Category
- Products
-
- FAQ
- Contact us
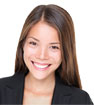
AC Drive Manufacturer
Variable Frequency Drive Installation Guide
Environmental Requirements
Most VFDs are supplied with a protection rating of IP20 (Finger proof). This normally requires that the variable frequency drive needs to be mounted in a floor standing enclosure or wall mounted panel, to increase the degree of protection.
Cooling
The reliable trouble-free operation of all industrial equipment is dependent upon operation in an environment for which that product was designed. The single most significant reason for the premature failure of a VFD controller is operation in excessive ambient temperature.
The design of the enclosure in which the VFD is housed is therefore of critical importance.
The following guide covers the basic calculations necessary to ensure that heat generated by a VFD can be satisfactorily transferred to the air surrounding the cubicle.
When making the calculation, remember to take account of all power dissipated inside the cubicle, and not simply that generated by the VFD. Further, in the internal layout of the cubicle, where possible, avoid placing electronic components at the top (hot air rises!), and where possible provide fans to circulate internal air.Remember, as a rule of thumb, an electronic product's lifetime halves for every 7°C temperature rise.
The enclosure itself transfers the internally generated heat into the surrounding air by natural convection, or external forced airflow. The greater the surface area of the enclosure walls, the better is the dissipation capability. Remember also, that only walls which, are not obstructed (not in contact with walls, floor or another hot enclosure) can dissipate heat to the air.
Calculate the minimum required unobstructed surface area Ae for the enclosure as follows:
To calculate the size of an enclosure to accommodate the following:
The minimum required unobstructed surface area Ae for the enclosure is as follows
If the enclosure is too large for the available space it can be made smaller by:
In this case the dimensions of the enclosure are determined only by the requirements to accommodate the equipment making sure to provide any recommended clearances. The equipment is cooled by forced airflow. This being the case it is important is such arrangement to ensure that the air flows over the heat-generating components to avoid localized hot spots.
The minimum required volume of ventilating air is given by:
Example
To calculate the size of an enclosure to accommodate the following guide:
Maximum dissipation of each EMC filter = 60W
Total dissipation = 3 x (570 +60) = 1890W
Then the minimum required volume of ventilating air is given by:
Electronic overload relays
It is important to recognize that the non-sinusoidal waveforms, and variable frequency, associated with variable frequency drives, invalidate the basis for protection afforded by most electronic overload relays. The use of such devices on the mains supply of a variable frequency drive is also invalid. Please consult with the manufacturer before using any of these devices.
Fusing
Fuses should not be seen as an overload protection device; variable frequency drives regulate the current flowing in the system and fusing needs to be designed to cater for catastrophic failure within the VFD or a short circuit between cables. High rupturing capacity fuses (HRC) act as clearing devices for sustained high currents and are consequently well suited to this type of duty, and is commonly recommended by most VFD manufacturers.
Typical fuse recommendations for three-phase variable frequency drives are given in Table 2.
Motor and variable frequency drive protection
Apart from the VFD built in motor overload protection, internal heat, short circuit and ground fault, protection is provided. The electronic overload protection for the motor also takes into account the reduced cooling when the motor is operated at low speeds without forced ventilation.
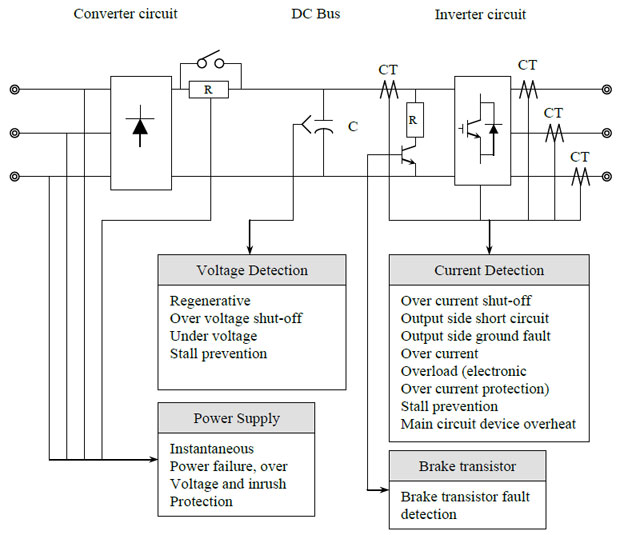
Long motor cables
The use of chokes to facilitate the application of long motor cables is rare. It is usually simpler and more elegant to use a larger variable frequency drive rating.
Because of charging currents, long motor cable runs can cause a reduction in the torque available from the variable frequency drive; this could also cause the variable frequency drive to trip due to excessive current normally experienced with smaller capacity variable frequency drives.
The following guidelines give suggested limits for standard shielded cables. The capacitance of the cable is approximately 300pF/m measured from the three phases together to the sheath. This is typical of steel wire armoured cable (SWA) and similar, where the individual phase conductors are surrounded by a further insulating medium before being covered by the screen. For other values of capacitance the length limits are approximately in inverse to the capacitance.
Note that individual separate phase conductors give lower capacitance, but may not be acceptable for EMC reasons. Cables where the screen is laid over the phase conductors and mineral insulated cables, are known to have much higher capacitance and should be treated with caution.
Maximum cable lengths at a specific supply voltage and switching frequency
The above cable lengths are for Gozuk VFDs. Please consult with the variable frequency drive Manufacturers before installing.
For other switching frequencies:
Output Chokes
Calculation of the necessary inductance of the choke is complex. However the following guidelines might be helpful.
Estimate the cable capacitance (C), from one line to all others.
Typical values
Maximum DC link voltage can be calculated from the highest r.m.s. AC supply voltage times 1.41
Now calculate the minimum inductance per phase from the following expression
The acceptable voltage drop at the working frequency determines the maximum value of inductance. Calculate this from the following expression:
If 2Lmin > Lmax then the variable frequency drive cannot operate with this length of cable and a higher rated variable frequency drive must be used.
Consideration must be given to the high frequency losses in the chokes. This can be estimated from the following expression:
It is now necessary to decide whether the choke is able to tolerate this loss. This is a difficult judgment. As a crude rule, the loss should not exceed 0.1 VA in the choke at maximum speed, i.e.:
Supply impedance
High quality commercial VFDs are designed to operate from typical industrial power distribution systems with a maximum fault level of ten to twenty times the variable frequency drive rated power. Problems can also occur if a VFD system is installed close to the main power supply or power factor correction capacitors, both of which present low supply impedance to the VFD. Variable frequency drives fitted wit D.C. link chokes are in general unaffected by low supply impedance.
A good rule of thumb is to ensure a total supply impedance of approximately 4 percent reactance. Where information about the supply is not known, it is good practice to fit line reactors of 2 percent.
There is no easy solution for high supply impedance as power lines carrying VFD current need to be oversized, as do transformers used in order to minimize the impedance. This over sizing may need to be as high as five times that normally considerate adequate.
Brake resistor selection
Firstly we need to understand what happens when a motor decelerates to stop under a high inertia load. When the motor starts to decelerate and the load keeps on rotating the motor, the motor starts to act as a generator sending energy back to the VFD.With energy from the main supply and from the motor, now acting as a generator,the DC Bus voltage level starts to rise from approximately 560VDC to levels that will activate a DC Bus over voltage trip. Most variable frequency drives can sustain levels up to 780VDC before tripping. Some variable frequency drives equipped with internal braking circuits and resistors will control this rise in DC Bus voltage but only for a short duration. If a lengthy or heavy-duty brake cycle is required, it is best to fit external units, which could cope with the extra energy safely.
To reduce the cost and physical size of VFDs, and the fact that very few applications require DC Bus braking, manufacturers only provide units with limited DC Bus braking capabilities. Users should consult with Manufacturers as to the capability of the units before installing them in these applications.
Two important factors to consider are the required braking torque and the duty cycle of the application.
Example:
A system inertia of 10kg m2 is to be decelerated from full load speed to rest. We need to find the braking resistor value and maximum braking torque
VFD rating = 30kW
Motor rating = 30kW
Motor full load speed = 1475rpm
Motor nominal torque rating = 191Nm
Repeat cycle time = 30 Seconds
Resistor operating voltage = 660V
Our first step is to determine the minimum deceleration time tb
Maximum braking torque Mb is identical to maximum accelerating torque
The motor is capable of delivering up to 150% of its full load rating for up to 60seconds maximum, i.e.:
As the braking operates intermittently, the resistor can be selected from a range offering "intermittent" rather than "continuous" power absorption. Advantage an also be taken of the overload rating of the resistor by applying an overload factor, which will be derived from a set of cooling curves obtained from the manufacturer or supplier of the resistor. In this example,deceleration time is taken as 7 seconds, repeat cycle time 30 seconds. From typical data the overload factor is 2.
The power rating of the chosen resistor is:
A braking resistor must be installed in accordance with instructions provided by its supplier or manufacturer. The braking resistor should incorporate a thermal tripping device, which should be connected, to a trip release mechanism to stop the VFD.
Resistors intended for braking duty should be capable of tolerating thermal shock "Pulse rated" resistors are recommended
Application selection
The following table is a guide of torque requirements in certain applications.
Most VFDs are supplied with a protection rating of IP20 (Finger proof). This normally requires that the variable frequency drive needs to be mounted in a floor standing enclosure or wall mounted panel, to increase the degree of protection.
Cooling
The reliable trouble-free operation of all industrial equipment is dependent upon operation in an environment for which that product was designed. The single most significant reason for the premature failure of a VFD controller is operation in excessive ambient temperature.
The design of the enclosure in which the VFD is housed is therefore of critical importance.
The following guide covers the basic calculations necessary to ensure that heat generated by a VFD can be satisfactorily transferred to the air surrounding the cubicle.
When making the calculation, remember to take account of all power dissipated inside the cubicle, and not simply that generated by the VFD. Further, in the internal layout of the cubicle, where possible, avoid placing electronic components at the top (hot air rises!), and where possible provide fans to circulate internal air.Remember, as a rule of thumb, an electronic product's lifetime halves for every 7°C temperature rise.
The enclosure itself transfers the internally generated heat into the surrounding air by natural convection, or external forced airflow. The greater the surface area of the enclosure walls, the better is the dissipation capability. Remember also, that only walls which, are not obstructed (not in contact with walls, floor or another hot enclosure) can dissipate heat to the air.
Calculate the minimum required unobstructed surface area Ae for the enclosure as follows:
Where:
Ae = Unobstructed surface area (m²)Example
P = Power dissipated by all heat sources in the enclosure (W)
Tamb = Maximum expected ambient temperature outside the enclosure (°C)
Ti = Maximum permissible ambient temperature inside the enclosure (°C)
k = Heat transmission coefficient of the enclosure material (Wm-2 ºC-1).
To calculate the size of an enclosure to accommodate the following:
- Two Gozuk 3.7kW VFDs
- EMC filter for each VFD
- Braking resistors mounted outside the enclosure
- Maximum ambient temperature inside the enclosure 40ºC
- Maximum ambient temperature outside the enclosure 30ºC
- Maximum dissipation of each VFD = 190W
- Maximum dissipation of each EMC filter = 25W
- Total dissipation = 2 x (190 + 25) = 430W
The minimum required unobstructed surface area Ae for the enclosure is as follows
If we select an enclosure with a height (H) of 2 m, a depth (D) of 0.6 m, and a minimum width W
Ae = 7.8m2
Dissipating surfaces Top + Front (Wmin x 0.6)+(Wmin x 2)+(2 x 0.6 x 2) Wmin |
> 7.8 m² > 7.8 m² > 7.8 m² > (7.8 – 2.4)/2.6 |
|
> 2.1 m |
If the enclosure is too large for the available space it can be made smaller by:
- Reducing the power dissipation in the enclosure
- Reducing the ambient temperature outside the enclosure
- Increasing the permissible ambient temperature inside the cubicle if possible by derating equipment in line with manufacturer's instruction
- Increasing the number of unobstructed surfaces of the cubicle
In this case the dimensions of the enclosure are determined only by the requirements to accommodate the equipment making sure to provide any recommended clearances. The equipment is cooled by forced airflow. This being the case it is important is such arrangement to ensure that the air flows over the heat-generating components to avoid localized hot spots.
The minimum required volume of ventilating air is given by:
Where:m3hr-1 (4)
V = Cooling air flow ( m3hr-1),Where:
P = Power dissipated by all heat sources in the enclosure (W),
Tamb = Maximum expected ambient temperature outside the enclosure (ºC),
Ti = Maximum permissible ambient temperature inside the enclosure (ºC) and
k = ratio of po / pi
po = Air pressure at sea level andTypically, a factor of 1.2 to1.3 can be used to allow for pressure drops in dirty air filters.
pi = Air pressure at the installation.
Example
To calculate the size of an enclosure to accommodate the following guide:
- Three Gozuk 15kW VFDs
- EMC filter for each VFD
- Braking resistors mounted outside the enclosure
- Maximum ambient temperature inside the enclosure 40º
- Maximum ambient temperature outside the enclosure 30º
Maximum dissipation of each EMC filter = 60W
Total dissipation = 3 x (570 +60) = 1890W
Then the minimum required volume of ventilating air is given by:
V = 737 m3hr-1
Electronic overload relays
It is important to recognize that the non-sinusoidal waveforms, and variable frequency, associated with variable frequency drives, invalidate the basis for protection afforded by most electronic overload relays. The use of such devices on the mains supply of a variable frequency drive is also invalid. Please consult with the manufacturer before using any of these devices.
Fusing
Fuses should not be seen as an overload protection device; variable frequency drives regulate the current flowing in the system and fusing needs to be designed to cater for catastrophic failure within the VFD or a short circuit between cables. High rupturing capacity fuses (HRC) act as clearing devices for sustained high currents and are consequently well suited to this type of duty, and is commonly recommended by most VFD manufacturers.
Typical fuse recommendations for three-phase variable frequency drives are given in Table 2.
Variable frequency drive rating | Recommended | ||
Power (kW) | Current (A) | Motor cable (mm²) | Fuse rating (A) |
0.75 | 2.1 | 1.5 | 6 |
1.5 | 3.8 | 2.5 | 10 |
2.2 | 5.6 | 2.5 | 10 |
4 | 9.5 | 2.5 | 16 |
5.5 | 12 | 2.5 | 16 |
7.5 | 16 | 4 | 20 |
11 | 25 | 4 | 35 |
15 | 34 | 6 | 40 |
18.5 | 40 | 10 | 50 |
22 | 46 | 10 | 60 |
30 | 60 | 16 | 70 |
37 | 70 | 25 | 80 |
45 | 96 | 35 | 100 |
55 | 124 | 35 | 125 |
75 | 156 | 50 | 160 |
90 | 180 | 70 | 200 |
110 | 202 | 95 | 250 |
Motor and variable frequency drive protection
Apart from the VFD built in motor overload protection, internal heat, short circuit and ground fault, protection is provided. The electronic overload protection for the motor also takes into account the reduced cooling when the motor is operated at low speeds without forced ventilation.
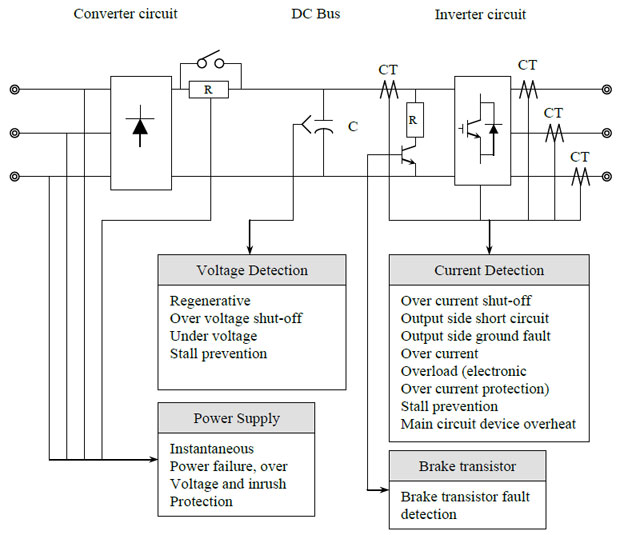
Long motor cables
The use of chokes to facilitate the application of long motor cables is rare. It is usually simpler and more elegant to use a larger variable frequency drive rating.
Because of charging currents, long motor cable runs can cause a reduction in the torque available from the variable frequency drive; this could also cause the variable frequency drive to trip due to excessive current normally experienced with smaller capacity variable frequency drives.
The following guidelines give suggested limits for standard shielded cables. The capacitance of the cable is approximately 300pF/m measured from the three phases together to the sheath. This is typical of steel wire armoured cable (SWA) and similar, where the individual phase conductors are surrounded by a further insulating medium before being covered by the screen. For other values of capacitance the length limits are approximately in inverse to the capacitance.
Note that individual separate phase conductors give lower capacitance, but may not be acceptable for EMC reasons. Cables where the screen is laid over the phase conductors and mineral insulated cables, are known to have much higher capacitance and should be treated with caution.
Maximum cable lengths at a specific supply voltage and switching frequency
Variable frequency drive Rating | Cable length (m) at 400V.A.C. supply voltage and 3kHz switching frequency |
0.75kW | 70 |
1.5kW | 100 |
2.2kW | 130 |
4kW | 200 |
5.5kW – 11kW | 250 |
15kW – 90kW | 300 |
The above cable lengths are for Gozuk VFDs. Please consult with the variable frequency drive Manufacturers before installing.
For other switching frequencies:
Maximum cable length = Maximum cable length at 3kHz x (3kHz / switching frequency)
Output Chokes
Calculation of the necessary inductance of the choke is complex. However the following guidelines might be helpful.
Estimate the cable capacitance (C), from one line to all others.
Typical values
Screened/Armoured cables where there is a plastic sheath between the phase and the screen: 130pF/mDecide on the available charging current
Screened cables with no plastic sheath between cores and screen, mineral insulated cables: 300pF/m
Add an allowance for the motor capacitance: 1nF is a reasonable estimate
Where:
In = Nominal rated r.m.s output currentMost variable frequency drives are rated at 150%, i.e. k = 1.5
k = Acceptable short term overload factor
Factor 1.41 = Maximum DC link voltage
Factor 2 = variable frequency drive instant trip current to nominal output current
Maximum DC link voltage can be calculated from the highest r.m.s. AC supply voltage times 1.41
Now calculate the minimum inductance per phase from the following expression
If using standard iron-cored chokes, the inductance at the high frequencies involved will be rather lower than the specified 50Hz inductance. A good rule of thumb is to specify an inductance of twice that determined by this calculation.
The acceptable voltage drop at the working frequency determines the maximum value of inductance. Calculate this from the following expression:
Where:
x = Acceptable volt drop over the chokeIf 2Lmin < Lmax then any value between these limits can be used.
VA.C. = Motor voltage rating (line to line r.m.s.)
f0 = Maximum variable frequency drive output frequency
If 2Lmin > Lmax then the variable frequency drive cannot operate with this length of cable and a higher rated variable frequency drive must be used.
Consideration must be given to the high frequency losses in the chokes. This can be estimated from the following expression:
Where:
fs = Switching frequencyThe factor 0.8 is a rough estimate of the fraction of the total losses dissipated in the choke. Note that the loss is proportional to the switching frequency so the lowest acceptable frequency should be selected.
It is now necessary to decide whether the choke is able to tolerate this loss. This is a difficult judgment. As a crude rule, the loss should not exceed 0.1 VA in the choke at maximum speed, i.e.:
Where:
fo(max) = Maximum output frequencyIf the loss exceeds this limit, and it is not possible to reduce the switching frequency, then a resistor should be connected in parallel with each choke to extract some of the power. The resistor value is given by:
The value is not critical and variations of ±50% are acceptable. The power rating of the resistor should beat least 0.8P. Provision must be made for the resistor to dissipate this power without overheating itself or nearby equipment. Values of 100W per phase are not uncommon.
Supply impedance
High quality commercial VFDs are designed to operate from typical industrial power distribution systems with a maximum fault level of ten to twenty times the variable frequency drive rated power. Problems can also occur if a VFD system is installed close to the main power supply or power factor correction capacitors, both of which present low supply impedance to the VFD. Variable frequency drives fitted wit D.C. link chokes are in general unaffected by low supply impedance.
A good rule of thumb is to ensure a total supply impedance of approximately 4 percent reactance. Where information about the supply is not known, it is good practice to fit line reactors of 2 percent.
There is no easy solution for high supply impedance as power lines carrying VFD current need to be oversized, as do transformers used in order to minimize the impedance. This over sizing may need to be as high as five times that normally considerate adequate.
Brake resistor selection
Firstly we need to understand what happens when a motor decelerates to stop under a high inertia load. When the motor starts to decelerate and the load keeps on rotating the motor, the motor starts to act as a generator sending energy back to the VFD.With energy from the main supply and from the motor, now acting as a generator,the DC Bus voltage level starts to rise from approximately 560VDC to levels that will activate a DC Bus over voltage trip. Most variable frequency drives can sustain levels up to 780VDC before tripping. Some variable frequency drives equipped with internal braking circuits and resistors will control this rise in DC Bus voltage but only for a short duration. If a lengthy or heavy-duty brake cycle is required, it is best to fit external units, which could cope with the extra energy safely.
To reduce the cost and physical size of VFDs, and the fact that very few applications require DC Bus braking, manufacturers only provide units with limited DC Bus braking capabilities. Users should consult with Manufacturers as to the capability of the units before installing them in these applications.
Two important factors to consider are the required braking torque and the duty cycle of the application.
Kinetic energy of the motor and load = 0.5 J ω2Where:
J = Total inertia (kg m²) of the motor, transmission and driven machineIf there is gearing between the motor and the driven machine, J is the value reflected at the motor shaft.
J = angular velocityAs the energy regenerated is proportional to the square of the angular velocity, most of the energy in the system is concentrated at the higher operating speed, and is delivered to the resistor at the start of the deceleration.
Example:
A system inertia of 10kg m2 is to be decelerated from full load speed to rest. We need to find the braking resistor value and maximum braking torque
VFD rating = 30kW
Motor rating = 30kW
Motor full load speed = 1475rpm
Motor nominal torque rating = 191Nm
Repeat cycle time = 30 Seconds
Resistor operating voltage = 660V
Our first step is to determine the minimum deceleration time tb
Maximum braking torque Mb is identical to maximum accelerating torque
But maximum deceleration occurs at 150% of motor nominal torque. The value to apply for Mb is thereforeNm
1.5 x 191 = 286.5 NmSo the actual deceleration time tb is:
This is the minimum deceleration time. For example, let us decide on a more practical deceleration time of 7seconds. We can now calculate the maximum braking torque to decelerate the load in 7 seconds:Seconds tb = 5.39 seconds
Braking Power is:Nm
=220.64 Nm
Resistor resistance value (Ω):=34.65 kW
The motor is capable of delivering up to 150% of its full load rating for up to 60seconds maximum, i.e.:
1.5 x30 = 45kW.It is therefore equally capable of regenerating the same short time power. As 45kW is in excess of 35kW required for the application, the value of the resistor is:
Resistor power rating Pr=12.45 Ω
As the braking operates intermittently, the resistor can be selected from a range offering "intermittent" rather than "continuous" power absorption. Advantage an also be taken of the overload rating of the resistor by applying an overload factor, which will be derived from a set of cooling curves obtained from the manufacturer or supplier of the resistor. In this example,deceleration time is taken as 7 seconds, repeat cycle time 30 seconds. From typical data the overload factor is 2.
The power rating of the chosen resistor is:
For practical purposes, it can be assumed that 15% to 20% of energy dissipated during the regenerative braking is due to electrical losses in the motor and variable frequency drive, and mechanical losses in the motor and load, all of which assist the braking. In practice, using the recommended resistor value will result in extra braking torque available. However, the rate of energy feedback from the load inertia is determined by the rate of deceleration.Pr = 35/2 =17.5kW
A braking resistor must be installed in accordance with instructions provided by its supplier or manufacturer. The braking resistor should incorporate a thermal tripping device, which should be connected, to a trip release mechanism to stop the VFD.
Resistors intended for braking duty should be capable of tolerating thermal shock "Pulse rated" resistors are recommended
Application selection
The following table is a guide of torque requirements in certain applications.
Name of Application | Load torque as percentage of full load driving torque | |||
Breakaway | Accelerating | Peak running | ||
Agitators | Liquid | 100 | 100 | 100 |
Slurry | 150 | 100 | 100 | |
Blowers, centrifugal | Valve closed | 30 | 50 | 40 |
Valve open | 40 | 110 | 100 | |
Blowers, positive-displacement, rotary, bypassed | 40 | 40 | 100 | |
Centrifuges (extractors) | 40 | 60 | 125 | |
Compressors, axial-vane, loaded | 40 | 100 | 100 | |
Conveyors, shaker-type (vibrating) | 150 | 150 | 75 | |
Escalators, stairways (starting unloaded) | 50 | 75 | 100 | |
Fans, centrifugal, ambient | Valve closed | 25 | 60 | 50 |
Valve open | 25 | 110 | 100 | |
Fans, centrifugal, hot gasses | Valve closed | 25 | 60 | 100 |
Valve open | 25 | 200 | 175 | |
Fans, propellers axial-flow | 40 | 110 | 100 | |
Frames, spinning, textile | 50 | 125 | 100 | |
Grinders, metal | 25 | 50 | 100 | |
Machines, buffing, automatic | 50 | 75 | 100 | |
Machines, cinder-block, vibrating | 150 | 150 | 70 | |
Machines, key seating | 25 | 50 | 100 | |
Machines, polishing | 50 | 75 | 100 | |
Mills, flour, grinding | 50 | 75 | 100 | |
Mixers, chemical | 175 | 75 | 100 | |
Mixers, concrete | 40 | 50 | 100 | |
Mixers, dough | 175 | 125 | 100 | |
Mixers, liquid | 100 | 100 | 100 | |
Mixers, sand, centrifugal | 50 | 100 | 100 | |
Mixers, sand, screw | 175 | 100 | 100 | |
Mixers, slurry | 150 | 125 | 100 | |
Mixers, solid | 175 | 125 | 175 | |
Pumps, adjustable-blade, vertical | 50 | 40 | 125 | |
Pumps, centrifugal, discharge open | 40 | 100 | 100 | |
Pumps, oil-field, flywheel | 150 | 200 | 200 | |
Pumps, oil, lubricating | 40 | 150 | 150 | |
Pumps, oil fuel | 40 | 150 | 150 | |
Pumps, propeller | 40 | 100 | 100 | |
Pumps, turbine, centrifugal, deep-well | 50 | 100 | 100 | |
Pumps, vacuum (paper-mill service) | 60 | 100 | 100 | |
Pumps, vacuum (other applications) | 40 | 60 | 100 | |
Pumps, vacuum, reciprocating | 150 | 60 | 150 | |
Rolls, crushing (sugarcane) | 50 | 110 | 125 | |
Rolls, flaking | 30 | 50 | 100 | |
Screens, centrifugal (centrifuges) | 40 | 60 | 125 | |
Screens, vibrating | 50 | 150 | 70 | |
Separators, air (fan-type) | 40 | 100 | 100 | |
Washers, laundry | 25 | 75 | 100 |
Post a Comment:
You may also like: